Is the next agricultural revolution inevitable?
What technological advances are needed to improve the efficiency of indoor farming?
Last time, we ruminated on the indispensability of natural sunlight in making controlled environment agriculture (CEA) feasible for nutrient-rich crops, such as wheat. We believe that in its current form (indoors with artificial light), VF (vertical farming) can only be widely adopted for low-calorie vegetables and leafy greens. In this article, we will examine energy losses at every stage of energy conversion, from light emission by LED to harvest, in an attempt to understand the reasons behind the low energy efficiency of photosynthetic food production.
Our goal is to speculate on potential ways in which the energy efficiency of the whole process might be improved, making it economically viable and sustainable for producing medium-calorie crops like potatoes or carrots through VF.
Although advances such as genetic engineering of photorespiration, manipulations with circadian clocks, light management, and LED efficiency enhancement are crucial for increasing energy efficiency, they will not be sufficient for the large-scale adoption of VF for some crops, such as rice or wheat. To combine efficient energy- and land use, we need a novel form of CEA that utilizes free sunlight. It appears quite plausible that energy-efficient CEA might become the basis of the next agricultural revolution.
Three phases of VF readiness
At the current stage of a hype cycle, investors become skeptical about VF. So did many scientists long before the downturn. Our previous article explains in detail why. But we are not as categorical as some skeptics.
We can estimate projected VF photosynthetic energy expenses for a number of crops just as we did for wheat in the previous article. Then we can compare this cost with corresponding market prices and range all crops into three categories, or phases of VF technology readiness (see the table below). Phase I crops have comparable electric energy costs to the current price. These crops are ready for adoption by the VF market now with minor technology adjustments and growth recipe optimization.
Phase II crops have an energy cost of 5 to ~ 10 times higher than the current price. VF of these crops is not viable now and significant breakthroughs are needed to make it work. Alternatively, phase II crops can be produced now in an economically viable way in CEA with solar light utilization.
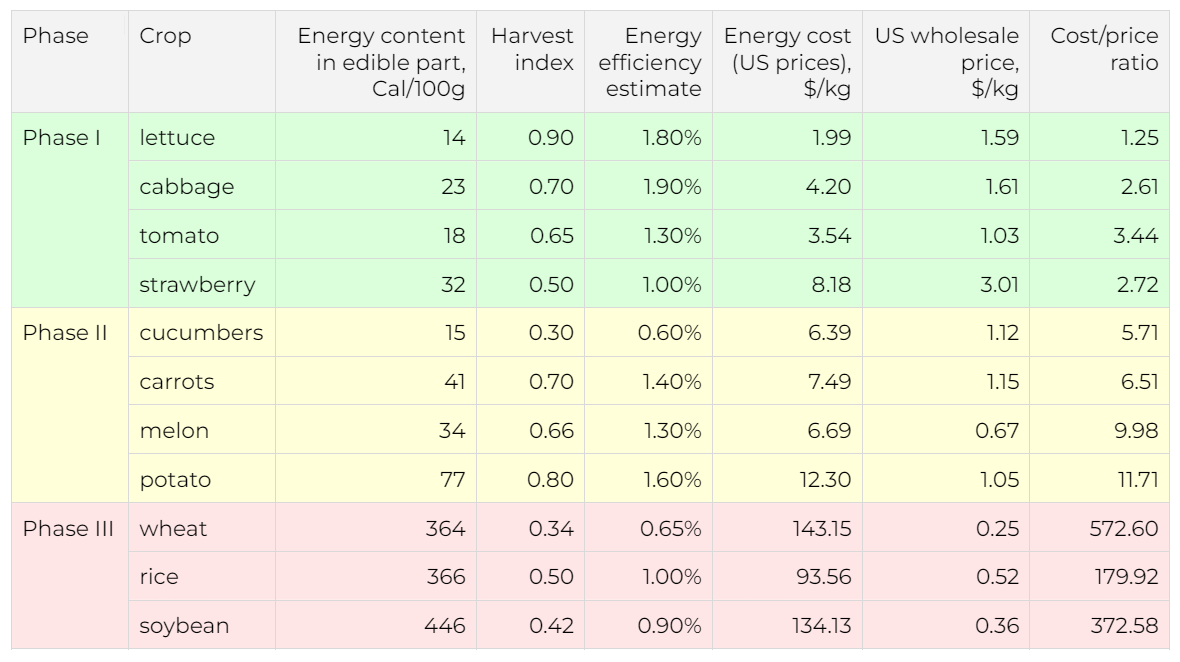
Phase III crops require so much energy that VF adoption does not seem like an option at this point. Even tremendous progress in light harvesting, which will include a combination of conceivable breakthroughs in green energy, LEDs, genetic engineering, and advances in CEA won’t be enough. But a combination of these breakthroughs and efficient sunlight utilization might bring these crops to some form of indoor agriculture in the future.
As progress accelerates, some crops will migrate from Phase II to Phase I category, or even from Phase III to Phase II. This already happens with tomatoes and strawberries. Essentially, there are two ways to accelerate this migration: increasing photosynthetic efficiency, and increasing light source cost efficiency. In today’s post, we will discuss disruptions that might enable this migration and thus potentially bring Phase II crops into the VF domain.
Energy transformations from LED to plant
There are many factors that determine the energy efficiency of crop harvesting. Each bar in the diagram below represents the energy left at the corresponding step as energy is inevitably lost at each step of transformations. Blue bars show the current state of affairs in energy loss at each step, something you can find in a textbook. And red bars are our estimates on how this might improve if realistic adjustments are made at each step. We start on the left with the energy consumed by the LED taken as 100% of the energy spent. The second step obviously represents losses to heat in the LED and ‘Direct light loss’ is about how much light ends up on a plant. These two parameters depend on light sources used for lighting and agrotechnical factors such as planting density, ability to focus light, etc. We will talk about them at the end of the article. Now, we will focus on photosynthetic efficiency, which is about the last five steps.
Photosynthesis hacking
Increasing photosynthesis efficiency includes playing with CO2 concentration, photoperiod, and fertilizer optimization which already is a routine for many CEA companies. For example, tomatoes need a four- to six-hour dark period at night to restore their photosynthetic ability. Lettuce, on the other hand, can be lit for 24 hours, requiring less light intensity than it would need in a natural environment. Circadian rhythms influence many processes, including nighttime carbon input for respiration and growth, flowering time, and many other agricultural characteristics. Fertilizers and pesticides are more effective when applied at certain times of the day.
Such factors have an effect mostly on the way light is absorbed by the leaf and the efficiency of capturing the absorbed light by chlorophyll. Finding the optimal recipe in terms of yield and energy consumption when there are many adjustable environmental parameters is an obvious task for ML techniques. Despite the plethora of scientific papers on this topic and significant progress made in recent years, there is still room for improvement. Based on the number of already published advances that are yet to be implemented on a wide scale, we expect an increase in the efficiency on the level of 10% both for light absorption and light collection by chlorophyll.
The photoperiod optimization, which is carried out in VF and CEA farms today, involves adjusting to the circadian rhythms of existing plants that were selected by breeders earlier. Early farmers seeking higher yields indirectly selected plants with optimized flowering time to account for temperature and humidity variations in an open field of their geography. In a closed environment, there is no more need to depend on daily and annual variations in temperature and illumination outside the farm building. The plant selection process itself can be carried out with an account of the requirement of the desired photosynthetic efficiency. This is the subject of chronoculture - altering the circadian rhythms of crops through gene selection and editing. The pervasive impact of the circadian system on crops suggests that future food production might be vastly improved by modifying circadian rhythms.
Knowledge of how the photoreaction unfolds over time can be used to hack photosynthesis at the next, carbohydrate synthesis stage when light is already captured by the chlorophyll. Some crops need short breaks to restore their photosynthetic ability. The light can be switched off during these breaks. Growing plants under pulsed light have been shown to reduce power consumption by more than 30% without compromising yields in some early experiments, and this seems to have a potential for further optimization.
Finally, the enzyme that is responsible for attaching the CO₂ molecule to the future sugar molecule can not only add carbon but is also less likely to add oxygen. As a result of such a side photorespiration reaction, instead of fixing carbon, the plant, on the contrary, loses it. It is one of the respiration processes that follow after carbohydrate synthesis. Existing genetic engineering solutions can suppress photorespiration and cause an efficiency increase of up to 40% and are on the way to commercialization by Living Carbon.
Further, genetic engineering undoubtedly will be the main tool for producing plants with a high harvest index (i.e., the ratio of edible mass to total plant mass, HI). High HI means greater efficiency in distributing nutrients produced in leaves into edible biomass. It is represented by the last step of energy conversion at the right of our diagrams.
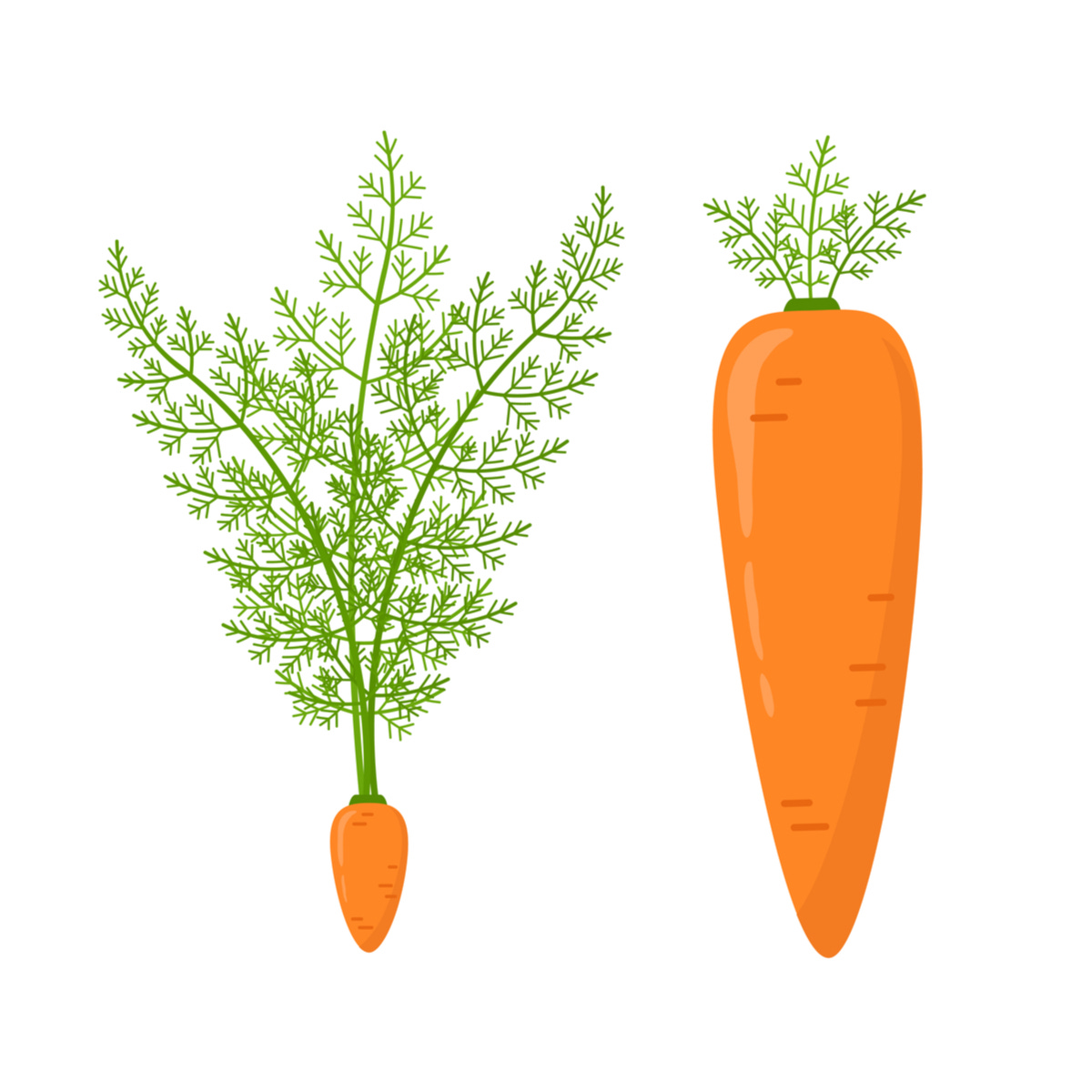
Let us return to wheat which has a relatively low HI. A significant part of the nutrients produced by photosynthesis is contained in stems, leaves, and roots that are not consumed by us. At the same time, the topmost leaf (known as the flag leaf) is denser and has a higher photosynthetic rate than other leaves, to supply carbohydrates to the developing seeds. The flag leaf, along with the second and third highest leaf on the plant, supplies the majority of carbohydrates in the grain. This example shows the pathway to increased harvest index: the selection of short plants with 2-3 topmost leaves. According to NASA’s results, a 100% increase in HI is already a reality for wheat. For plants with already high HI, like carrots or potatoes, HI is close to its limit of 1, so these speculative projections in our diagrams should of course be adjusted when considering a particular plant.
Energy-efficient light management
Last but not least, the way we convert electric energy into usable light is a step where cost reduction is possible. It is about, for example, the LED efficiency that had impressive progress in the past two decades. Although there is still some room for a further increase, LEDs are already quite efficient, and we obviously can’t go beyond 100% efficiency (that would mean either power generators instead of LEDs or a violation of the law of energy conservation). We assume that a 25% increase from the current ~44% energy efficiency to nearly 55% is possible within the next decade. Emulating sunlight spectrum or in general LED spectrum engineering, is also an important part of the race for energy-efficient light harvesting since the early NASA experiments.
It is necessary to look for other methods of energy saving. For example, it is possible to focus the light on germinating plants, instead of illuminating the soil of the garden bed. This will help to eliminate direct light losses. As the plant upgrows, such focusing becomes no longer necessary, as well as the required photon spectrum may change in response to biochemical processes change. The photoperiod and other external conditions (temperature, humidity) may also be altered in a course of plant growth. After all, free natural sunlight can be used on the upper floors of vertical farms, which is already being done by some players in the market. Given the value of direct losses (half energy lost at this conversion step), we speculate that a 50% efficiency increase is possible at light management.
All these adjustments to the plant lifecycle might lead to a lot of additional capital costs. But there might be ways to mitigate that, for example, instead of varying light and temperature conditions around a given plant, it is reasonable to divide the farm into various zones and transport trays with the plants through zones in a conveyor fashion.
Here we come to a VF-greenhouse hybrid where each farm layer is a separate climate zone. There are existing proposals that are designed for improving the energy efficiency of Phase I crops. As the upper layer blocks the light for the bottom layers, growing Phase-III crops in such hybrids will not be energy-efficient enough.
We believe that the future of Phase II crops (and, tentatively, Phase III crops) lies in the development of such concepts. Sunlight can and should be effectively redistributed from the upper to the lower floors. Surely this is not a problem of cutting-edge science. For example, one can use solar concentrators or light guides (as is already done in architecture). After all, in some regions, even the top layer needs just 50% sunlight in summer, the rest of the light simply turns into heat or dries the plants unnecessarily. Sunlight management is a promising avenue to explore in farming.
A new agricultural revolution is inevitable
Multiplying all the discussed increments in the energy conversion chain, we arrive at the overall increase of 825% in the efficiency of converting electricity into food (the utilization of free sunlight is not accounted for). This means that the energy efficiency of wheat harvesting will increase from 0.65% up to 5.4%. All this seems to be overly complicated: too many conditions to satisfy and scientific disruptions to happen. But this is how progress works. To allow you reading this text on your way home using your smartphone, a lot of things had to happen since 1990, crazy things at that time: the internet, mass distribution of cell phones, inconceivable miniaturization of integrated circuits, OLED displays with a touch-screen, and many more. Note that we have not accounted for an electricity cost drop of ~70% that will be caused by the green energy transition. Altogether, photosynthesis hacking, light management, automation, and green energy cost decrease can enable CEA adoption of all Phase-II crops that we have talked about today.
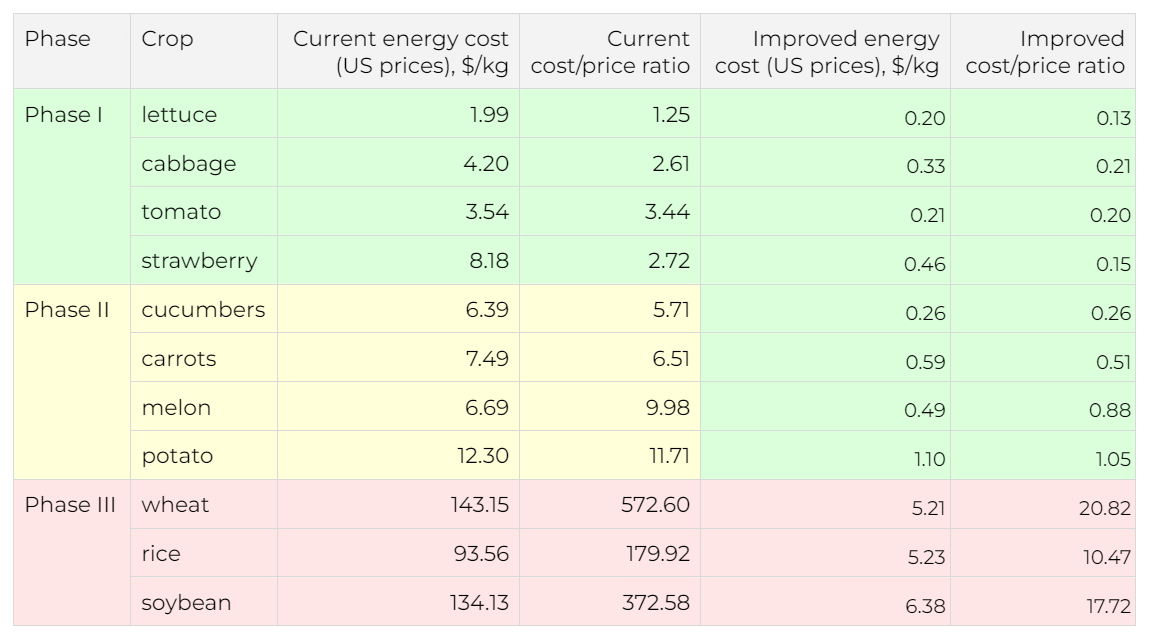
Disruptions are accelerating, so the CEA of many (if not most) crops will happen in one or another way. We believe that a new, Fourth Agricultural Revolution is inevitable. To be prepared, investors have to dive deep into the science of what’s happening. In Tidal Wave we do!
Thank you for breaking this down!